GOGOA Revolutionizes Neuroscience: Central Pattern Generator-Based Controllers Redefine Mobility Assistance
- Gogoa Mobility Robots
- Feb 22, 2024
- 4 min read
In an exciting step towards more effective and personalized neurorehabilitation, Gogoa Mobility Robots is on the verge of changing the landscape with its innovative development based on the theory of Central Pattern Generators (CPGs).
These CPGs, described as neural networks that endogenously generate rhythmic patterns, represent a significant milestone in the understanding and application of neuroengineering to improve mobility in patients with neurological injuries.
Picture this: at the beginning of the robotic neurorehabilitation process, when you engage with an exoskeleton, you're following a predetermined gait pattern, a sort of guide for your movements. But then, as your walking ability improves and your gait becomes more natural, that's when the GCP-based controllers come into play. This innovative control system adapts to your unique gait pattern, inspired by the natural movement of your body, providing personalized and precise assistance tailored to your specific needs.
Having GCP-inspired control represents a revolutionary advancement in applied neuroengineering. Although this development has not yet been integrated into HANK, Gogoa Mobility Robots' flagship exoskeleton, it is expected to be soon, taking robot-assisted rehabilitation to a new level of effectiveness and personalization.
The scientific foundation behind CPGs dates back over 50 years, when they were discovered in invertebrate animals by pioneering researchers like Wiersma, Hughes, and Wilson in the 1960s. Subsequent studies revealed that these neural generators are capable of producing coordinated motor patterns without relying on external sensory inputs, challenging previous conceptions about the need for proprioceptive feedback for the generation of complex movements.

Figure 1. Coordinated motor outputs in leg nerves for swimming in the abdominal nerve chain of the crayfish. A. Three cycles of motor outputs from the pair of legs on the left and right sides of an abdominal segment. B. Eight simultaneous recordings showing two cycles of firing in axons innervating four pairs of legs in four abdominal segments. (Mulloney and Smarandache, 2010)
The demonstration of "fictive motor patterns" in invertebrate preparations was crucial in understanding the endogenous nature of rhythmic pattern generation. This phenomenon, where motor neurons generate activity similar to what they would produce in vivo even in the absence of sensory inputs, provided solid evidence of the autonomy of CPGs in producing coordinated movements.
The initial surprise at the discovery of CPGs lay in the prevailing belief that the generation of complex motor patterns required peripheral sensory information. However, further research revealed that these neural generators are capable of handling a variety of rhythmic behaviors, including locomotion, swimming, and breathing, in both invertebrates and mammals.
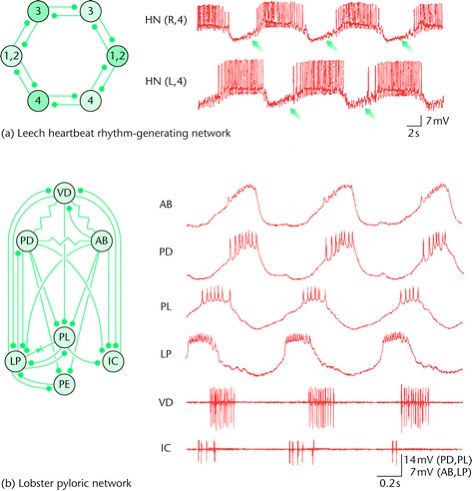
Figure 2. Mechanisms of rhythmicity in the central pattern generator. a) Network-based rhythmicity (heartbeat rhythm generator of the leech). The network (top left) consists of a ring of six interconnected neurons with reciprocal inhibitory synapses. Neuron pairs 3 and 4 are particularly important as each forms a medio-central oscillator. Neurons in each pair discharge in antiphase (top right), while neurons 1 and 2 form a coordinated coupling between the medio-centers of neuron 3 and neuron 4; the network produces a two-phase pattern in which opening and closing neurons discharge in antiphase (left). Arrows indicate slow depolarization that allows the off-neuron to escape inhibition. Modified from Marder and Calabrese (1996). b) The pyloric network of the lobster is driven by an endogenous oscillatory neuron. Left, the complement of the neuronal network and synaptic interconnectivity (inhibitory synapses, stick-ball connectors; electrical coupling, resistors; rectifying electrical coupling, diode). Neuron AB is an endogenous oscillator and in most cases is the rhythmic driver of the network. Input to neuron AB induces post-inhibitory rebound and plateau in its follower neurons and as a consequence of these effects and interactions between follower neurons, the network produces a multifase rhythmic neural output (right). Neurons, VD, ventricular dilator; PL, late pyloric; PE, early pyloric.
The application of this knowledge in clinical settings has revolutionized the rehabilitation of neurological injuries, especially in patients with spinal cord injuries. CPGs offer a new perspective on movement generation, showing that neural rhythmicity can be induced through interactions between neurons in neural networks or through interactions between ionic currents in individual neurons.
Understanding the mechanisms underlying rhythmic pattern generation has led to the development of computational and experimental models that have revealed the complexity and flexibility of CPGs in producing multiple output patterns. This versatility offers promising potential for personalizing mobility assistance in neurorehabilitation, dynamically adapting to the individual needs and capabilities of each patient.
The integration of GCP-inspired controls into mobility assistance devices such as Gogoa Mobility Robots' HANK exoskeleton represents an exciting step forward in the practical application of this groundbreaking theory. By offering personalized and adaptable assistance based on each individual's unique gait pattern, GCPs are poised to transform the way neurological rehabilitation is approached, paving the way for more effective and satisfying recovery for patients.
For more information on the scientific basis behind this development, you can refer to the following links: http://www.facmed.unam.mx/Libro-NeuroFisio/FuncionesGenerales/CPGs/CPGs.html
In summary, Central Pattern Generator-based controllers represent a crucial innovation in neurorehabilitation, based on decades of scientific research that has revealed the intrinsic ability of the nervous system to generate coordinated movements. With their clinical application and integration into mobility assistance devices, GCP-based controllers promise to significantly improve the quality of life for patients with neurological injuries, offering hope and opportunities for more complete and functional recovery.
-
Central Pattern Generators Controllers by GOGOA
留言